LabVIEW 2018 Real-Time Module Help
- I want to change priority PERMANENTLY to REAL TIME but this is not working. Realtime value is 4 as far i know. Using this will result in process being set to normal priority. This is how to save priority permanently, BUT it works only with: low. Lower then normal. Above normal. IT DOES NOT WORK WITH: realtime.
- Never do this. Under any circumstances. As far as I am aware, input devices use priority level 15 (non-realtime critical) together with device drivers and the like.
The priority only dictates how often the OS should try to run your process. But take a look in task manager when playing a game your game already takes 99% of the CPU time (if it was badly coded). So no matter how high priority you set, you'll never be able to achieve more than a 1% speedup.
March 2018Part Number: 370715P-01
»View Product Info
DOWNLOAD (Windows Only)
LabVIEW 2015 Real-Time Module Help |
LabVIEW 2016 Real-Time Module Help |
LabVIEW 2017 Real-Time Module Help |
LabVIEW 2018 Real-Time Module Help |
LabVIEW 2019 Real-Time Module Help |
This topic explains how the LabVIEW Real-Time Module handles priorities and execution systems when creating and scheduling threads. By understanding the priority-based scheduling model, you can leverage the strengths of the LabVIEW Real-Time Module to create deterministic applications.
Threads, Priorities, and Execution Systems
LabVIEW is a structured dataflow language that abstracts many of the underlying complexities of computer operation to create an intuitive graphical programming environment. To understand how LabVIEW schedules parallel tasks based on priority, it helps to define some key concepts that underlie the scheduling process in LabVIEW:
- Thread—The basic unit of concurrency in the LabVIEW Real-Time Module. Operations within a thread execute sequentially, while each thread executes independently.
- Scheduler—An algorithm the operating system uses to schedule threads. The scheduler determines which thread to run on each CPU in the system at any given time.
- Run Queue—The queue of threads ready to be scheduled.
- Preemptive Scheduling—The process whereby the scheduler interrupts a lower-priority thread as soon as a higher-priority thread is ready to run.
- Round-Robin Scheduling—The process whereby the scheduler switches periodically between threads of equal priority to promote equal sharing of processor time.
- Execution System—A pool of related threads. Use the Execution page of the VI Properties dialog box to configure the execution system of a VI.
- VI Priority—The priority you assign to a VI on the Execution page of the VI Properties dialog box.
- Timed Loop Priority—The priority you assign to a Timed Loop using either the Priority input or the Configure Timed Loop dialog box.
- OS Priority—The priority assigned to a thread by the real-time operating system. LabVIEW assigns each thread an OS priority based on the priority of the containing VI or Timed Loop.
- Time-Critical—The highest priority available for a LabVIEW VI. Time-critical threads run above the priority of the scheduler itself. Therefore, time-critical threads always run to completion or until blocked.
- Priority Inheritance—The process whereby a thread temporarily assumes the priority of the calling thread. Priority inheritance helps to prevent priority inversions.
- Jitter—A measure of the extent to which execution timing fails to meet deterministic expectations.
Mpeg4 converter mac. The following illustration summarizes the priorities and execution systems available in LabVIEW.
The preceding illustration shows the following facts:
- Execution systems are independent of priority levels. LabVIEW uses execution systems only as thread pools and does not prioritize threads based on execution system. For each execution system, LabVIEW creates up to four threads at each priority level. This rule extends to each core in a multi-core system, so on a dual-core system, LabVIEW could create up to eight threads at each priority level for each execution system. Because the number of threads per execution system is limited, balancing your execution system assignments can be important. If you assign too many VIs to the same execution system, the VIs must share threads, limiting the potential parallelism of the VIs.
- Timed structure priorities fall between the high and time-critical VI priority levels.
- When you place parallel code inside a single Timed Structure, LabVIEW serializes the code because each timed structure comprises one and only one thread.
- LabVIEW Real-Time Module systems include many system threads running in parallel to the threads that comprise your LabVIEW VIs. These system threads execute at various priorities. For example, the NI Scan Engine thread, when present, runs by default at a priority above time-critical.
Priority Inheritance
LabVIEW implements priority inheritance to prevent unintended priority reductions. If a subVI is configured with a lower priority than that of the calling VI and both run within the same execution system, the subVI inherits the priority of the calling VI. If a subVI is configured with a priority higher than that of the calling VI, the subVI maintains its configured priority.
Note If a subVI runs in a different execution system than the calling VI, priority inheritance does not occur. |
The real-time operating system (RTOS) also implements priority inheritance. https://downzfile209.weebly.com/bettertouchtool-2-685.html. When a lower-priority thread holds a shared resource needed by a higher-priority thread, the RTOS temporarily increases the priority of the thread holding the shared resource so that the resource can return promptly to the higher-priority thread.
Time-Critical Priorities
There are two types of time-critical threads:
Real Time Priority For Games
- VIs set to time-critical priority—VIs set to time-critical priority run above the priority of the scheduler itself. Therefore, VIs set to time-critical priority always run to completion or until blocked, typically by the inclusion of a timing function or a function that waits for an external event.
- Timed Structures—A Timed Structure can be interrupted by another Timed Structure of higher priority or by a VI set to time-critical priority.
Note National Instruments recommends creating no more than one time-critical task per CPU. |
Timed Structure Priorities
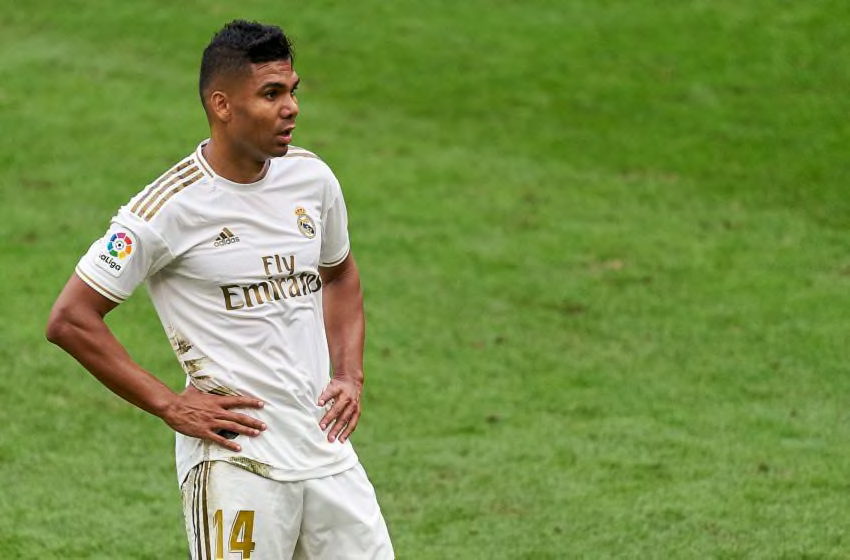
LabVIEW uses two separate but related priority schemes: VI priorities and timed structure priorities. Timed structure priorities are numeric, and a higher value represents a higher priority relative to other timed structures executing on the target. However, all timed structure priorities fall between the high and time-critical VI priority levels.
Note(Real-Time Linux) Do not use more than 32 timed structure priority levels in a VI. LabVIEW returns an error if you attempt to set more than 32 timed structure priority levels. |
Avoiding Errors When Using Priority Schemes
National Instruments recommends using only one priority scheme in your application, as shown in the following examples:
- If the application uses timed structures, keep all VIs at normal priority.
- If the application uses VIs set to time-critical priority, do not place timed structures inside the VIs set to time-critical priority.
Using a single priority scheme makes the application easier to understand and less error prone.
Scheduling
Scheduling is the process of determining which task to run at a given time. Scheduling is a key task for any modern operating system, but especially for a real-time operating system (RTOS). In addition to threads spawned by your VIs, an RT target runs numerous OS and driver threads at various priorities. To ensure that your tasks execute according to your timing requirements, it helps to understand how LabVIEW Real-Time schedules threads.
Basic RT Scheduling Rules
The following rules summarize the operation of the LabVIEW Real-Time scheduler when a new thread enters the run queue:
- Perform preemptive scheduling among threads of different priority.
- Perform round-robin scheduling among threads of equal priority.
- Time-critical threads always run to completion.
Understanding How LabVIEW Real-Time Module Scheduling Works
At any given time, each thread in the system is either running or blocked:
- Running—The thread is in the run queue, which means it is either ready to execute or is currently executing.
- Blocked—The thread cannot execute until some event occurs, such as the completion of an I/O operation or a timing function.
When a thread begins executing, it runs until one of the following conditions arises:
- The thread becomes blocked by a Wait VI within a While Loop, the built-in timing mechanism of a Timed Loop, or a blocking function such as the Read Variable with Timeout function.
- The thread is interrupted by a higher-priority thread.
- The thread finishes executing.
When a thread becomes unblocked, it enters the run queue. The run queue is always sorted in priority order, so when a thread enters this queue, it immediately moves ahead of all lower-priority threads. If a thread entering the run queue is higher-priority than a currently-running thread, the higher-priority thread either runs on a different CPU core or interrupts the currently-running thread in a process called preemptive scheduling.
Preemptive Scheduling
Preemptive scheduling is the process of ordering thread execution based on the relative priorities of the threads. When a thread of higher priority than the current thread enters the run queue, the scheduler interrupts the current thread if necessary so that the higher-priority thread can execute immediately. The interrupted thread then returns to the run queue behind the higher-priority thread.
Round Robin Scheduling
Round robin scheduling is the process of alternating between threads such that each thread receives roughly equivalent CPU time. During round robin scheduling, the scheduler interrupts and switches between the threads at regular intervals, keeping track of the CPU time given to each thread.
The LabVIEW Real-Time Module performs round robin scheduling among threads of equal priority. However, the LabVIEW Real-Time Module does not perform round-robin scheduling among VIs set to time-critical priority.
Setting Loop Priorities Based on Timing Requirements
Although it seems intuitive to set the priority of each loop based on the perceived importance of the loop, this strategy can cause jitter. Set the priority of each loop not based on how critical the task is within the application but rather based on how important it is that the task fulfill a certain timing guarantee. For example, in a data logging application with a data acquisition loop and a logging loop, you might be tempted to make the logging loop the higher-priority loop. However, data acquisition must occur at regular intervals to avoid missed data points. However, it does not matter when you log each data point to disk, as long as you log each point eventually. In this case, you should assign a higher priority to the data acquisition loop, even though data logging is the primary purpose of the application.
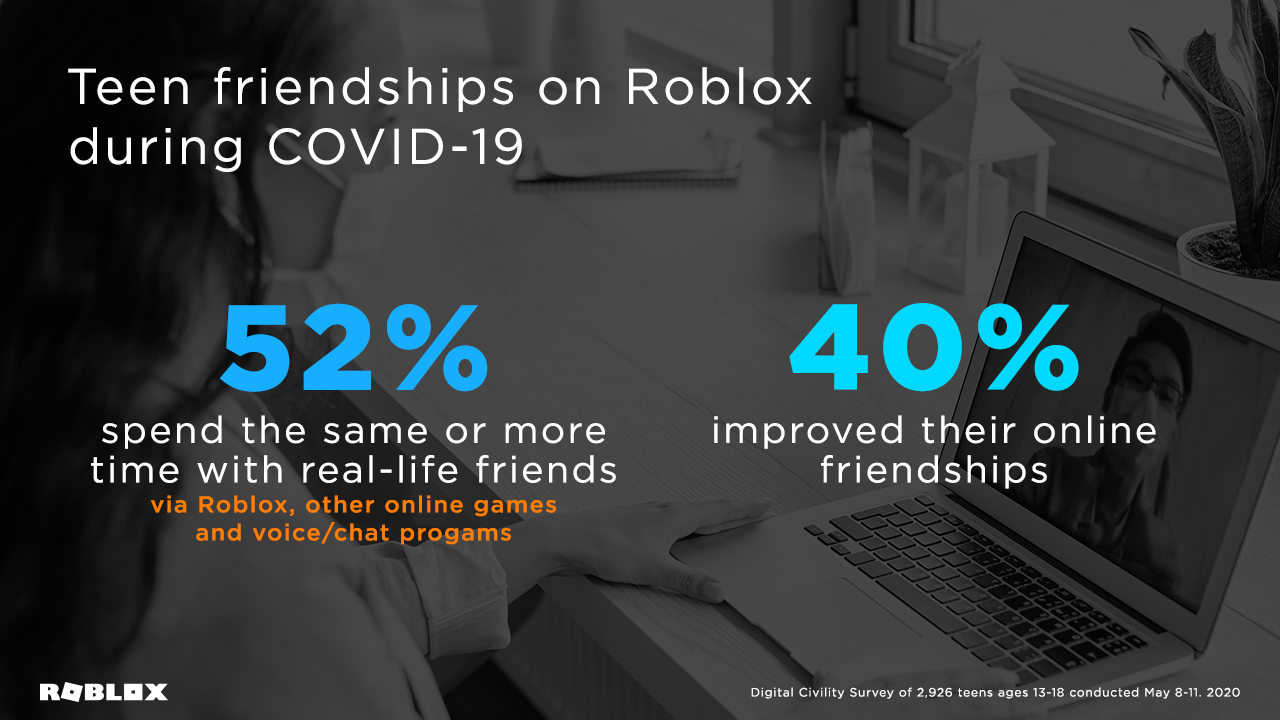
LabVIEW uses two separate but related priority schemes: VI priorities and timed structure priorities. Timed structure priorities are numeric, and a higher value represents a higher priority relative to other timed structures executing on the target. However, all timed structure priorities fall between the high and time-critical VI priority levels.
Note(Real-Time Linux) Do not use more than 32 timed structure priority levels in a VI. LabVIEW returns an error if you attempt to set more than 32 timed structure priority levels. |
Avoiding Errors When Using Priority Schemes
National Instruments recommends using only one priority scheme in your application, as shown in the following examples:
- If the application uses timed structures, keep all VIs at normal priority.
- If the application uses VIs set to time-critical priority, do not place timed structures inside the VIs set to time-critical priority.
Using a single priority scheme makes the application easier to understand and less error prone.
Scheduling
Scheduling is the process of determining which task to run at a given time. Scheduling is a key task for any modern operating system, but especially for a real-time operating system (RTOS). In addition to threads spawned by your VIs, an RT target runs numerous OS and driver threads at various priorities. To ensure that your tasks execute according to your timing requirements, it helps to understand how LabVIEW Real-Time schedules threads.
Basic RT Scheduling Rules
The following rules summarize the operation of the LabVIEW Real-Time scheduler when a new thread enters the run queue:
- Perform preemptive scheduling among threads of different priority.
- Perform round-robin scheduling among threads of equal priority.
- Time-critical threads always run to completion.
Understanding How LabVIEW Real-Time Module Scheduling Works
At any given time, each thread in the system is either running or blocked:
- Running—The thread is in the run queue, which means it is either ready to execute or is currently executing.
- Blocked—The thread cannot execute until some event occurs, such as the completion of an I/O operation or a timing function.
When a thread begins executing, it runs until one of the following conditions arises:
- The thread becomes blocked by a Wait VI within a While Loop, the built-in timing mechanism of a Timed Loop, or a blocking function such as the Read Variable with Timeout function.
- The thread is interrupted by a higher-priority thread.
- The thread finishes executing.
When a thread becomes unblocked, it enters the run queue. The run queue is always sorted in priority order, so when a thread enters this queue, it immediately moves ahead of all lower-priority threads. If a thread entering the run queue is higher-priority than a currently-running thread, the higher-priority thread either runs on a different CPU core or interrupts the currently-running thread in a process called preemptive scheduling.
Preemptive Scheduling
Preemptive scheduling is the process of ordering thread execution based on the relative priorities of the threads. When a thread of higher priority than the current thread enters the run queue, the scheduler interrupts the current thread if necessary so that the higher-priority thread can execute immediately. The interrupted thread then returns to the run queue behind the higher-priority thread.
Round Robin Scheduling
Round robin scheduling is the process of alternating between threads such that each thread receives roughly equivalent CPU time. During round robin scheduling, the scheduler interrupts and switches between the threads at regular intervals, keeping track of the CPU time given to each thread.
The LabVIEW Real-Time Module performs round robin scheduling among threads of equal priority. However, the LabVIEW Real-Time Module does not perform round-robin scheduling among VIs set to time-critical priority.
Setting Loop Priorities Based on Timing Requirements
Although it seems intuitive to set the priority of each loop based on the perceived importance of the loop, this strategy can cause jitter. Set the priority of each loop not based on how critical the task is within the application but rather based on how important it is that the task fulfill a certain timing guarantee. For example, in a data logging application with a data acquisition loop and a logging loop, you might be tempted to make the logging loop the higher-priority loop. However, data acquisition must occur at regular intervals to avoid missed data points. However, it does not matter when you log each data point to disk, as long as you log each point eventually. In this case, you should assign a higher priority to the data acquisition loop, even though data logging is the primary purpose of the application.
What are the options for real-time scheduling?
A number of scheduling concepts have been developed for implementation in a real-time operating system (RTOS). The most commonly encountered is the pre-emptive scheduler even though it is not inherently a real-time algorithm in contrast to, for example, deadline scheduling, which aims to ensure that critical threads are executed within a given timeframe.
Desktop operating systems are designed around the concept of fairness – that no application should be starved of processing cycles by another. These systems tend to use round-robin scheduling, in which each task will run for a set period of time before being forced to yield access to the processor so that execution can switch to a different task that is ready to run. Once all tasks that are not blocked from running have been allotted a timeslice, execution resumes with the first task and the cycle continues.
In a real-time system, it is generally acceptable to starve less important tasks of processor cycles if there are critical tasks with work to do – although determining how ‘unimportant' a task really is can be problematic for guaranteeing overall system stability.
How does the typical scheduler operate?
The simplest possible scheduler conceptually is the main()
loop – it simply cycles through a series of functions. As long as the critical functions execute within the maximum allowable processing latency of the system, the loop will provide satisfactory performance. However, every logical task within the system is provided with the same execution priority and will consume processor cycles even if they have no work to do. It becomes very difficult to guarantee that the loop will finish execution within the maximum allowable latency for all situations. Applications also become difficult to maintain beyond a certain size. At this point, it makes sense to break the application down into discrete tasks and use an RTOS scheduler to control their execution.
Disclosure settle zip. A pre-emptive RTOS works on the basis that the task with the highest priority and which is ready to run will be the one that is scheduled for execution. Typically, the RTOS will examine the list of tasks after any change of task status – usually after a system call or an interrupt. For example, a task may relinquish control of a mutual-exclusion semaphore (mutex) on which a higher-priority task is blocked. The RTOS will note that the high-priority task is now ready to run and pick it for scheduling. That task will continue execution until it is replaced by a higher-priority task, yields the processor, or becomes blocked again. Because the task can remain running, it is possible that it could starve other tasks of execution time – a risk that system designers need to take into account. Conversely, the RTOS guarantees that the most critical thread that is ready to run will be able to access the processor as soon as it requires it.
What are the common pitfalls in scheduling?
In principle, it is possible to analyze a system for potential scheduling problems and to ensure that the system will meet its deadlines. However, the analysis is greatly complicated by any interprocessor communication. Basic rate-monotonic analysis, one of the earlier theories used for determining schedulability – and the subject of one of the 20 most commonly cited papers in computer science – can only guarantee schedulability for tasks that do not share resources. In practice, most systems demand shared access to memory objects and peripherals that make schedulability, as well as the tendency to deadlock, difficult to predict.
One problem encountered with conventional pre-emptive RTOS schedulers is that of priority inversion. In this situation, a low-priority task obtains access to a shared resource but is pre-empted by a higher priority task, blocking all other tasks that need that resource. If a critical task requires that resource, it cannot run until the low-priority task has released the mutex. But until activity has subsided far enough to allow the low-priority task to run, it will be unable to continue far enough to release the mutex. During this time, the effective priority of the critical task is reduced to that of the low-priority thread: hence priority inversion.
Real Time Priority For Games On
One workaround, although it can introduce other schedulability problems if implemented without safeguards, is to use the priority-inheritance protocol. This mode provides any thread that owns a mutex with the same priority as a more important task that is blocked on it until the semaphore is released.
Real Time Priority For Games Today
Many RTOS implementations support priority inheritance or a close relative of the technique, the priority ceiling protocol, which prevents a low-priority task from being elevated to the highest possible priority in the system. There are dangers in using the protocol: designers need to ensure that a normally low-priority task will not simply hog a resource and keep running indefinitely in a state in which it cannot easily be pre-empted.
There also also subtleties in implementation. If an application is moved from a single-core to a dual-core processor that uses the priority-ceiling protocol, it cannot guarantee mutual exclusion. So a distributed priority ceiling protocol has to be used instead.
Because of the problems of analyzing schedulability in asynchronous, interrupt-driven real-time systems, many systems that have to guarantee dependable behaviour resort to some form of strict time-sharing. In this scenario, important tasks are guaranteed a number of cycles within a period of time to run, even though they have nothing to do, just in case they do need to respond to a problem. ARINC 653 avionics systems have used this approach for years and a number of automotive systems have adopted the Flexray architecture, which is based on a similar time-triggered approach.
Each partition in an ARINC 653 system has its own dedicated, protected memory space and each partition can run a multitasking system. Vital functions usually have dedicated partitions. Even with such rigidly enforced partitions, timing problems can still arise through interactions with hardware. One problem that has been identified in a paper by GE Aviation and Wind River Systems lies in the use of direct memory access (DMA). If a partition towards the end of its time-slice decides to initiate a long DMA transfer, the partition that runs immediately afterwards can stall because the DMA hardware has exclusive access to the memory bus – effectively shortening the new partition's timeslice and creating the potential for it to miss its own deadline.
The recommendation in this case is to transfer the responsibility for setting up DMA transfers to a system-level task that takes into account the amount of time a partition has remaining before it is forced to relinquish the processor.
Similarly, interrupt handling can upset the operation of an otherwise strictly time-sliced system. A number of systems prevent all but the system timer, which is used to help manage scheduling, from being able to assert an interrupt. Others may record the interrupt and then allow the affected system to poll for the associated data when it next runs.